The Revolution of 3D-Printed Organs: A Journey from the 1980s to Today
Introduction
3D bioprinting is a rapidly evolving technology that enables the fabrication of complex biological structure, like tissues and organs, by precisely layering bioinks composed of living cells and biomaterials. This technology holds immense potential in regenerative medicine, organ transplantation, tissue engineering, drug testing, and disease modelling.
The concept of bioprinting has been developed by the traditional 3D printing, since 1980s from a niche prototype technology. It is also known as additive manufacturing. Using a computerized design produced with computer-aided design (CAD) software, it builds an object by applying successively thin layers of a material, such as plastic, metal, resin, or even biomaterials. The method starts with slicing the digital 3D model into multiple thin layers. The 3D printer then follows these instructions, carefully depositing material layer by layer to gradually produce the physical thing. This technology has been expanded into various industries, includes automobile, medical, dental, aerospace and consumer goods.
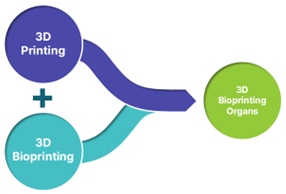
Fusion of 3D Printing and Bioprinting
Applications of 3D-bioprinting in healthcare:
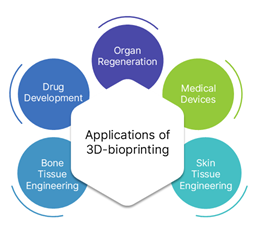
3D-printed organs are biologically, functionally accurate copies of actual bodily parts. They are created using cellular bio-inks and are cultured artificially in a laboratory. 3D bioprinting techniques have developed as a versatile tool in tissue engineering and regenerative medicine for creating or patterning functional 3D biostructures with precise geometric shapes, bridging the gap between designed and natural tissue constructs.
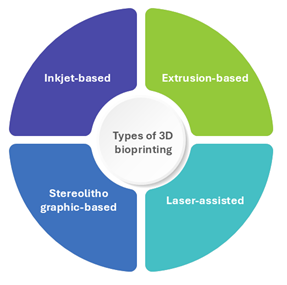
Different types of 3D bioprinting techniques
Inkjet-based bioprinting: Inkjet bioprinting is considered the first bioprinting technique when Elmqvist of Siemens patented the first practical inkjet device in 1951. Inkjet printing takes a ‘bottom-up’ method to structure fabrication rather than the traditional ‘top-down’ technique. Inkjet printing is also known as droplet-based printing because the ink is jetted through the nozzle of a jetting equipment in the form of droplets with nano-to pico-litre volumes. The mechanism involved in it is deposition of tiny bio-ink droplets onto a surface using thermal, acoustic, or piezoelectric forces.
Inkjet printing involves two steps:
- The creation of discrete droplets that are directed to a specific area on the substrate.
- The interaction between droplets and the substrate.
Extrusion based bioprinting: Extrusion-based bioprinting (also known as direct ink writing), which evolved from inkjet printing, is the most popular method of 3D bioprinting due to its versatility and low cost. Instead of a single droplet, extrusion-based bioprinting generates continuous filaments by extrusion force. This method can be used to print biomaterials with a variety of viscosities and cell concentrations.
Laser-assisted bioprinting: This approach, also known as Laser Induced Forward Transfer, utilizes a pulsed laser beam to deposit bio-ink onto the substrate. The use of a laser for placing cells permits non-contact 3D printing of organs and tissues. This technique consists of three essential components: a bio-ink-coated ribbon, a pulsed laser source, and a substrate on which the bio-ink will be deposited.
Stereolithographic based bioprinting: SLA is the first commercial 3D printing method, developed by Charles W. Hull in 1984. He developed 3D structures by scanning a vat of light-sensitive material with UV radiation and curing it layer by layer. Because of its high resolution, SLA is frequently used in bioprinting to create precise tissue scaffolds with adjustable geometry and porosity structure. Boland’s team at Clemson University first used a commercial SLA printer to implement cell-loaded SLA printing in 2004. Following that, several research groups optimized SLA technology, resulting in its further utilization in bioprinting. Currently, SLA technology has been used mostly in scaffold printing rather than cell-loaded bioprinting.
Pros & Cons of 3D-printed organs:
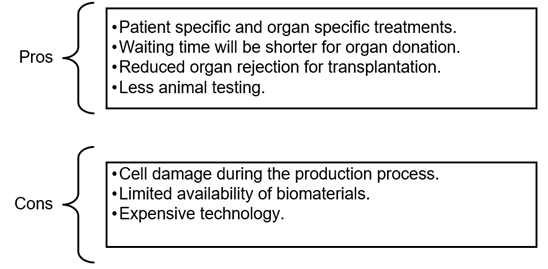
A few milestones- 3Dprinted organs

Bioprinting and Intellectual Property: Emerging Patent Trends
Leading countries, like US (United States), CN (China), EP (European Patent Office), JP (Japan), KR (South Korea), IN (India), and CA (Canada), are actively publishing patents and driving innovations in bioprinting of organs and including WO (WIPO), patents filed through the WIPO’s Patent Cooperation Treaty (PCT) will enter the national phase in designated countries following the international phase, allowing applicants to seek protection in individual jurisdictions. These nations are at the forefront of advancing 3D bioprinting technologies, focusing on regenerative medicine, tissue engineering, and organ fabrication.
Among the key players in the market, Organovo Inc., Cellink Co. Ltd., and Revotek Co. Ltd. stand out as prominent assignees, consistently filing patents and pioneering breakthroughs in bioprinting. Their innovations are shaping the future of personalized medicine, organ transplantation, and biomedical research, reinforcing the growing importance of intellectual property in this rapidly evolving field. Additionally, leading inventors in this field are making significant contributions, Gatenholm Erik, Martinez Hector, and Presnell Sharon C.
Conclusion
3D bioprinting has evolved as a revolutionary method that has the potential to revolutionize tissue engineering, organ transplantation, and medicine. Significant advancements have been made in bioprinting over the years, from early studies with inkjet-based bioprinting to more sophisticated methods including extrusion, laser-assisted, and stereolithographic bioprinting. Initially, scientists worked on printing basic tissue shapes, but developments in stem cell integration, vascularization methods, and bioink formulations have made it possible to create more intricate, useful tissues. Today, researchers are making progress in printing completely functional, transplantable organs, and bio printed skin, cartilage, and organoids are already being utilized for regenerative medicine, drug testing, and research. Even while there are still obstacles to overcome, such as scalability, regulatory approval, and ethical issues, the advancements in recent years are undoubtedly encouraging.